It is impossible to overstate the impact and influence of carbon dioxide on all aspects of plant growth. Carbon alone accounts for 45% of the dry weight of any plant. Only oxygen has an equal standing at 45%, with hydrogen at 6% completing the top three elements required for a plant to survive. Carbon is a nutrient, just as surely as nitrogen is a nutrient, but since it is derived from the air, it is sometimes overlooked.
Carbon comes in a convenient plant-available form, carbon dioxide (CO2), a gas. Plants have become excellent assimilators of carbon used as the simple building blocks that they need to grow and reproduce. Any discussion surrounding carbon dioxide should begin with an understanding of carbohydrates: what they are, and how they are made.
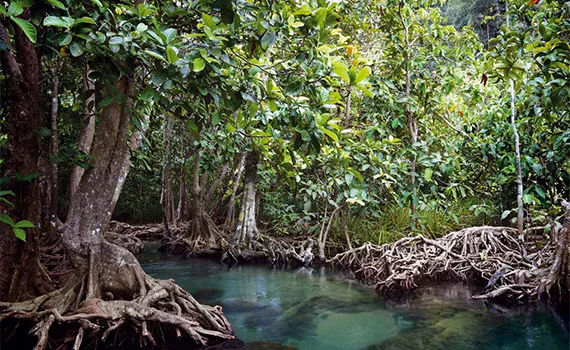
The Product
Carbohydrates are the basic building blocks of all things classified as life. Some life forms produce carbohydrates, some life forms consume carbohydrates. Carbohydrates are a group of organic compounds that contain only carbon, hydrogen and oxygen usually in a 1:2:1 ratio. They include sugars, starches, cellulose, and many others. They are the major energy source for animals and are produced by photosynthesis in plants. Animals obtain their carbon by consuming carbon as carbohydrates and proteins, while plants get their carbon by assimilating a gas, CO2. There are two basic processes that occur to produce carbohydrates in a plant: the first is what is known as the light reaction and the second the dark reaction.
Both occur in an organelle in plant cells called the chloroplast. The light reaction is, as the name implies, the reaction that occurs in the presence of light photons and converts light energy into chemical energy, ATP (Adenosine-5’-triphosphate) and NADPH (Nicotinamide adenine dinucleotide phosphate). These energy molecules are then used in the dark reaction (more appropriately called the carbon reaction) to produce triose phosphate, the basic carbohydrate. In reality, the dark reaction still requires light to function, not only to produce energy, but for many other light-influenced controls. This dark reaction is known as the Calvin Cycle.
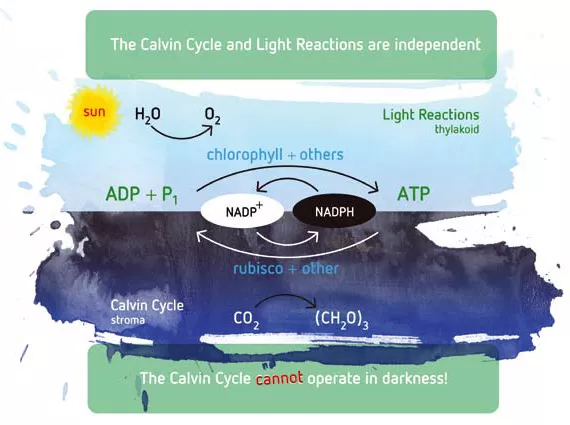
In this cycle, a base carbohydrate is used as a seed molecule (known as a substrate molecule) with 5 carbons to capture CO2 with the help of a light-activated enzyme known as Rubisco that also adds water molecules and is ultimately split into 3 carbon molecules that undergo more changes to become a carbohydrate, triose phosphate. The problem is:
- 85% of the base carbohydrates produced return to the system; and
- Oxygen (O2) is more attractive than CO2 and destroys the seed molecules stopping the process; this is known as photorespiration.
The Process
Air from around the leaves enters the leaf tissue through specialized pores known as stomata. Once inside, the molecules in the air will dissolve into the fluid around the inner plant cells, mesophyll cells, and then on through the cell membranes, across the cytosol, and to the chloroplast in this liquid phase. From the moment the CO2 approaches the stomata, it has to go through several areas of resistance including the boundary at the opening of the stomata, the interior air boundaries including the stomatal cavity, and the intercellular air space. This resistance is caused by the lack of movement in the air, meaning that molecules like CO2 have to diffuse slowly across the opening of their own accord.
Gas molecules will disperse quickly when there are currents of air, but in the calm stillness of a boundary, they have to move under their own vibration or the action of another molecule – a very slow process. Once in a liquid state, the CO2 molecules then have to diffuse across and through the cells until they can be integrated into the Calvin Cycle. The problem remains that there is much more oxygen than carbon dioxide in the air, so the O2 is moving right along with the CO2 but at a higher concentration.
Once they become part of the Calvin Cycle, there will be more O2 available than CO2, unless the concentration is increased or the Calvin Cycle is isolated into areas from which oxygen can be excluded or limited. The process we have described so far is both the basic idea in the conversion of light energy into carbohydrates, and the exact description of one of the three types of metabolism plants employ to do this. This one is known as the C3 cycle (also known as C-3 metabolism); it is the preferred cycle of most dicots but some monocots as well.
The next cycle is the C4 cycle, so named because it captures the CO2 in a 4-carbon sugar in the mesophyll cells, and transports it to the chloroplasts in bundle sheath cells to be processed in the Calvin Cycle, thus limiting the amount of oxygen in the process. C4 plants include all angiosperm plants and most monocots such as grass, maize, and sugar cane. C4 plants do better in hot dry locations because the process that causes the photorespiration mentioned earlier increases with temperature. Both C3 and C4 cycles require light to function. There is one more C4 variant which performs part of the cycle in daylight followed by another part during the night. These are known as CAM (crassulacean acid metabolism) (see figure 3).
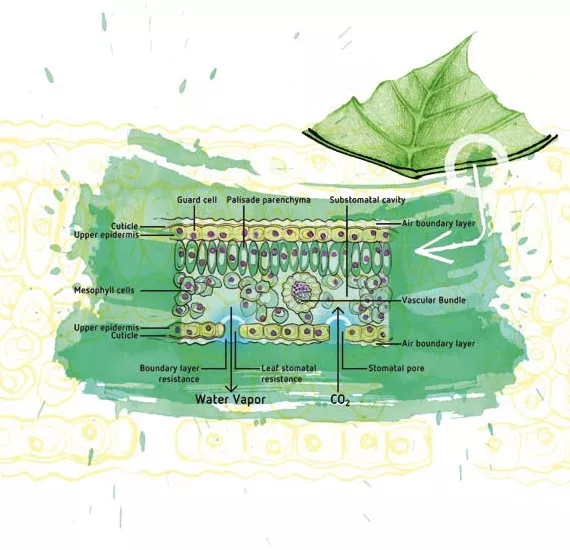
Carbon dioxide needs to be allowed in, to make carbohydrates and to do this the stomata have to open. Once they are open, not only can CO2 enter, but water can escape, and so limiting the opening time of the stomata to the hours of darkness is a clear evolutionary advantage. There is one other important note here, C3 plants work better at higher concentrations of CO2 whereas C4 plants do better at lower concentrations of the gas because they actively pump the gas into the cycle.
The Environment
In plants where the CO2 moves into the Calvin Cycle of its own accord (C3), the concentration of CO2 in the cell will pretty much equal the atmospheric concentration. A growth increase of 30 – 60% has been documented when CO2 is supplied at 600 – 700 ppm in C3 plants. C4 plants, on the other hand, had no such advantage because in these plants the CO2 is actively captured, transported, and concentrated at the same rate, no matter what the atmospheric concentration is. The ideal CO2 level for C4 plants is the naturally occurring ambient level. So some plants do respond to higher levels of CO2, but photosynthesis reaches a maximum rate (saturation) at a given point, and going higher than this is pointless. At really high levels above 3% or 3000 ppm, the rate falls off. The ideal level for C3 plants seems to be 600 – 700 ppm; any more than this will not produce any additional benefit so it will be a waste of money. Keep in mind that this level is what should be available around the stomata.
The boundary resistance we mentioned earlier applies to the area immediately adjacent to the stomata opening. When the air is still, the air under or next to leaves and inside the physical structure of the plant will have lower levels of CO2 and O2 than the air because the plant is removing these elements. This air will also be more humid because the plant is transpiring to cool itself and transport nutrients from the root system. In short there is a microclimate right next to the plant’s leaves.
The lower levels of CO2 will mean that less is available in the cells and photosynthesis will slow down. The higher humidity in the air means that less water can evaporate out of the plant and so there will be less movement of water through the plant. By creating a movement of air, we can make sure that more of the necessary components come close to the stomata, while unnecessary components like water are dispersed.
Putting this into Practice
Clearly, the CO2 cycle in the plant is part of other systems and affected by many factors including temperature, light, and many more. The movement of CO2 is only a small part of what is actually going on. We have sought to simplify a very complex process, but along the way, we have uncovered some very important information.
There are differences in the ways that plants capture carbon dioxide. When CO2 is limited, so is the production of substrates. CO2 is only used during the light cycle, even though some plants can capture it at night to use during the day. Temperature has been shown to be an issue especially at upper ranges. Certain plants (C3) benefit from higher ambient levels of CO2 while for others (C4 and CAM) adding CO2 is a waste of resources. There are many barriers that the CO2 molecule has to cross to become part of the Calvin Cycle. Air movement at all levels is critical to eliminate the micro-climate associated with leaf surfaces.
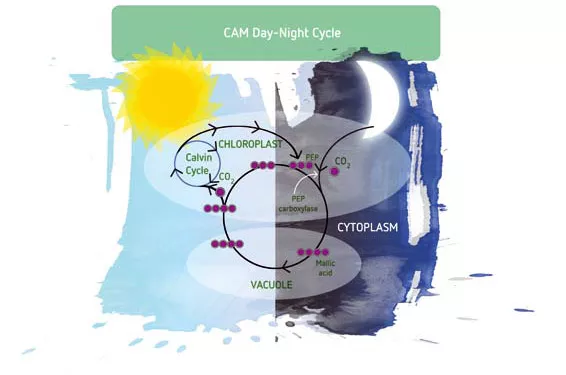
Now, how can we make this information work for us?
Outdoor crops and indoor crops have different capabilities. Outdoors, little can be controlled and crops are basically at the mercy of nature. Indoors, the restrictions of technology and resources apply. The benefits of air movement apply to all plants, so this is the first thing to look at. For indoor plants, fans are essential to move the air inside the growing area, as well as to exchange the old air for CO2 refreshed air. For outdoor plants, increasing the spacing between plants will help control some micro-climate formation, or at least its severity. Enriching the air with CO2 in covered crops (indoor or tented) is an option, but is almost useless for C4 plants.
If a grower has C3 plants and can control the atmosphere, then he or she can add extra CO2. There are two basic ways to apply CO2. The first is to use bottled CO2 gas released through a regulation system and the second is a burner system, whereby gas is burned in the room to produce CO2 as a by-product. Both methods have drawbacks. Bottled systems require many bottles and frequent trips to have them refilled. Gas burners are less expensive and require less effort; however burners can malfunction causing a range of issues including safety.
Temperature affects photosynthesis. In indoor crops, the plants need light to function but lighting can increase temperature. Throw a CO2 burner into the mix and overheating can rapidly become an issue. Venting to the outside and drawing in fresh air will take away all the CO2 that has been applied. It is pointless to apply CO2 during the dark when things are cooler because it will not work. So what can be done? Well, it really depends on the money. If the money is there, first arrange for good air movement in the growth zone of the plants in the room, use air conditioning in the room or water chiller units and leave the room closed, switch lights to sealed air-cooled lights, use bottled CO2, or vent when needed and replace the CO2. Any of these measures will work but some are better for hotter environments.
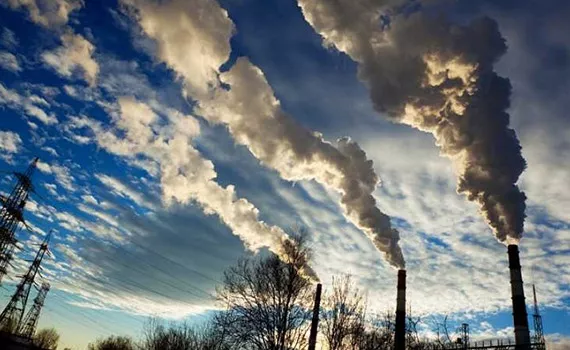
During periods of high heat stress, the crop can be saved by shading or switching off lights. CO2 use will also fall off so stop supplying it. Watch the humidity because plants can go a long way in cooling themselves when moisture conditions are right for good water movement. However, there needs to be enough water available at all times for this, otherwise leaf wilting and tissue damage can result. Too much water, however, will slow down transpiration enough to reduce the cooling effect. Above all, balance the system by using workable set-points and automated controls when working out the enrichment times and ventilation. Always calibrate the monitoring equipment.
Carbon-skinned balloon
In the end, plants need carbon dioxide. The gas becomes the plant in every way, like a carbon-skinned water balloon. Plants obtain the carbon they need in different ways, or rather the same way with an extra step or two thrown in. Because of the botanical differences, some plants do well with higher concentrations of ambient CO2 while others will be indifferent. Growth can be affected positively by managing the CO2 as a ‘nutrient’. It is possible to benefit from the introduction of additional CO2, but all other factors for growth – such as temperature and humidity – also have to be taken into account.
Basically a plant is a kind of carbonaccumulating machine. As such, carbon dioxide’s role in plant growth cannot be overstated.